Researchers in Texas showed strong results in their efforts to develop artificial muscles, engineers in St. Louis devised a mostly painless alternative to getting blood drawn, and MIT scientists turned to artificial intelligence in hopes of predicting future cancer. Also in this week’s coolest scientific discoveries: a quicker flight to Mars?
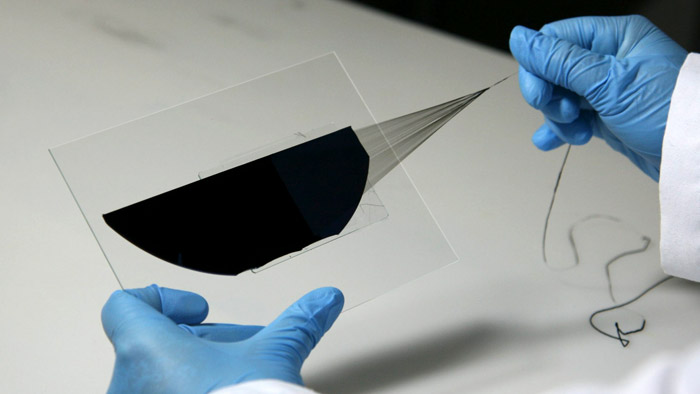
What is it? Researchers at the University of Texas at Dallas are developing electrochemically driven carbon nanotube (CNT) muscles that could have applications “from robotics and heart pumps to morphing clothing.”
Why does it matter? Previously researchers focused on thermally powered muscles, made of twisted or coiled carbon nanotube or polymer yarns, which could contract when heated and return to their original length when cooled. But that technology had limitations, requiring, for instance, large amounts of input energy. As the authors of the new study explain in Science, “Success in making artificial muscles that are faster and more powerful and that provide larger strokes would expand their applications.”
How does it work? The electrochemically powered CNT yarn muscles are “actuated by applying a voltage between the muscle and a counter electrode, which drives ions from a surrounding electrolyte into the muscle,” according to UT Dallas. The interior surfaces of the coiled CNT yarns are also coated with a special polymer that contains either positively or negatively charged particles, said chemistry professor Ray Baughman. “This polymer coating converts the normal bipolar actuation of carbon nanotube yarns to unipolar actuation, where the muscle actuates in one direction over the entire stability range of the electrolyte,” Ray Baughman said. “This long-sought behavior has surprising consequences that make electrochemical carbon nanotube muscles much faster and more powerful.”
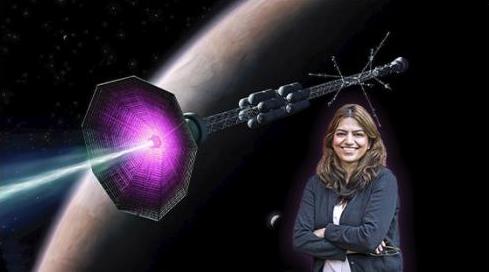
What is it? Plasma — electrically charged gas that’s considered the fourth state of matter — is at the heart of a new rocket thruster design that could propel spacecraft to Mars and beyond.
Why does it matter? Earthlings have got their eyes on the red planet. NASA’s Artemis program, for instance, aims to put humans back on the moon by 2024 and then use it as a jumping-off point for trips to Mars. Even when they’re lined up closest, though, the minimum distance between Earth and Mars is about 34 million miles, so it’s a bit of a trip. Technology like the plasma-propelled engine conceived by Princeton Plasma Physics Laboratory’s Fatima Ebrahimi — who describes it in a paper in the Journal of Plasma Physics — could speed things up a bit.
How does it work? Currently “space-proven” plasma thrusters use electric fields to propel particles of plasma out the back of a rocket. But Ebrahimi’s advance relies on a process called magnetic reconnection, “in which magnetic fields converge, suddenly separate and then join together again, producing lots of energy,” according to PPPL. Ebrahimi said, “Long-distance travel takes months or years because the specific impulse of chemical rocket engines is very low, so the craft takes a while to get up to speed. But if we make thrusters based on magnetic reconnection, then we could conceivably complete long-distance missions in a shorter period of time.”
Ingenuity That’s Only Skin-Deep
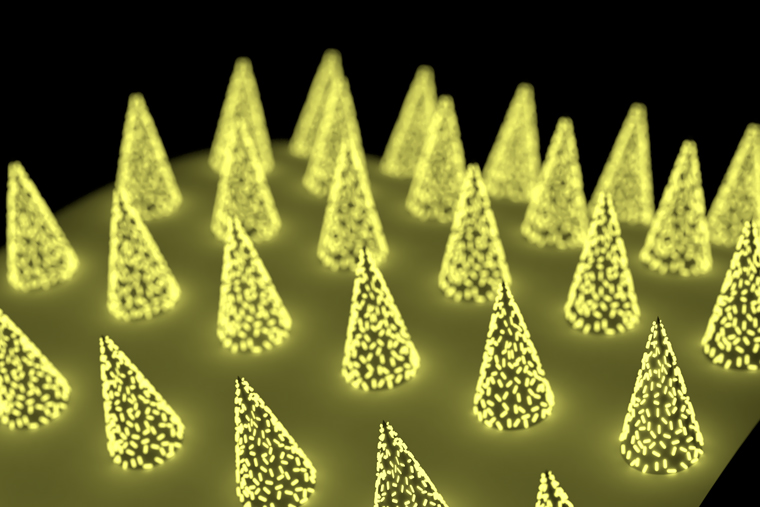
What is it? Scientists at the McKelvey School of Engineering — part of Washington University in St. Louis — developed a microneedle patch that can be applied to the skin and detect certain biomarkers, such as for viral or bacterial infections or conditions like sepsis. No syringes required, and it’s “nearly pain-free.”
Why does it matter? In addition to sidestepping the hassle of getting blood drawn, the low-cost patches could speed up the diagnostic process. EMTs in an ambulance could apply a patch to somebody with chest pain, for instance, to test for a biomarker that would indicate myocardial infarction, rather than waiting to get to the hospital, draw blood there and send it to the lab. Patients with chronic conditions could also apply the patches to themselves for routine testing, rather than having to go to the hospital each time.
How does it work? The patches detect biomarkers that exist not only in blood but in the dermal interstitial fluid that surrounds our cells, where the biomarkers exist in lower concentrations. The use of plasmonic fluors — an “ultrabright fluorescence nanolabel” — facilitates the detection of the biomarkers even at low concentrations in the fluid, with the patch penetrating only about 400 microns deep into the dermal tissue. The technology is described further in a new paper in Nature Biomedical Engineering.
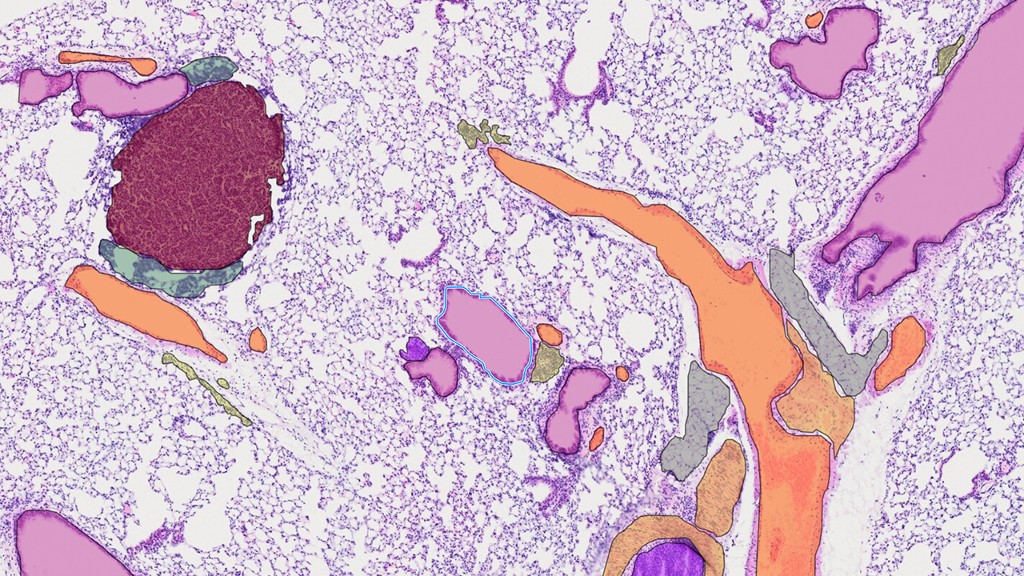
What is it? A consortium of European hospitals, research centers and pharmaceutical companies is looking to develop better healthcare artificial intelligence by compiling the largest database of pathology images.
Why does it matter? Called BIGPICTURE, the six-year, 70-million-euro project is being coordinated out of Radboud University Medical Center in the Netherlands. In a news release, Radboud notes that “pathology is the cornerstone” of how diseases are understood, how surgeons follow up after transplants and how drugs are evaluated for safety. But it “still relies heavily on the qualitative interpretation by a qualified pathologist.” Computers can help.
How does it work? Indeed they already are. In recent years, healthcare systems have turned to AI for assistance — advanced algorithms can quickly sift through reams of medical scans, for instance, sussing out patterns and learning to spot abnormalities. The more information AI is trained on, the more helpful it can be. BIGPICTURE aims to collect an initial set of 3 million digital slides from humans and lab animals, after first creating infrastructure to store and process all that information and formulating legal and ethical guidelines for its use.
What is it? Here’s an example of AI’s healthcare potential: Researchers at MIT developed “Mirai,” a system that can help predict future risk of breast cancer based on a patient’s mammograms.
Why does it matter? “Improved breast cancer risk models enable targeted screening strategies that achieve earlier detection, and less screening harm than existing guidelines,” said Adam Yala, a doctoral student at MIT’s Computer Science and Artificial Intelligence Laboratory (CSAIL) and the lead author of a new paper in Science Translational Medicine. “Our goal is to make these advances part of the standard of care.”
How does it work? The latest advances build on ongoing work undertaken by CSAIL and MIT’s Jameel Clinic. Two years ago, the team introduced a version of the algorithm that sought to erase diagnostic disparities between white and Black patients, which has hindered other efforts to use AI in medicine. The model still needed improvement, though, so the researchers trained it on a data set of 200,000 exams from Massachusetts General Hospital, then validated it on test sets from around the world. Now installed at Mass General, Mirai “jointly models a patient’s risk across multiple future time points and can optionally benefit from clinical risk factors such as age or family history, if they are available,” according to MIT News.