Researchers made colors disappear, turned common red bricks into batteries, and granted the senses of sight and touch to a nonhuman system. Was it magic? Nope: science! Read on for this week’s coolest discoveries.
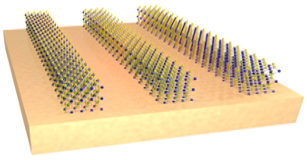
What is it? “Intrinsic color” — the kind we usually perceive — is created by different wavelengths of light being absorbed by the atoms, molecules, and surface structures that make up whatever we’re looking at. But now engineers at the University of Pennsylvania have designed a “system of nanoscale semiconductor strips” that makes the intrinsic color of a material disappear.
Why does it matter? According to a Penn Engineering news release, the new system — described in an article in Nature Communications — could have uses in “holographic displays and optical sensors,” and could “pave the way for new types of microlasers and detectors, fundamental elements of long-sought-after photonic computers.”
How does it work? The strips take advantage of so-called structural color. One example of structural color is peacock feathers, which have no one intrinsic color. The birds’ brilliant plumage is the effect of different wavelengths reflecting off the nanoscale structures on their feathers’ surfaces, colliding and interfering to create that iridescent sheen. The Penn researchers designed their nanoscale strips from tungsten disulfide on a gold backing. At only a few dozen atoms thick, the strips “are spaced out at suboptical wavelength sizes, allowing them to give off the type of structural color” exemplified by peacock feathers. In this way the strips, which should look blue, appear to have no color at all. If biological materials like feathers can have little to no intrinsic color but appear colorful due to their nanoscale structures, lead researcher Deep Jariwala said, this study suggests the reverse is also true: “If a material does have a strong intrinsic color, we show that one can do the opposite and make it disappear with appropriate nanostructuring.”
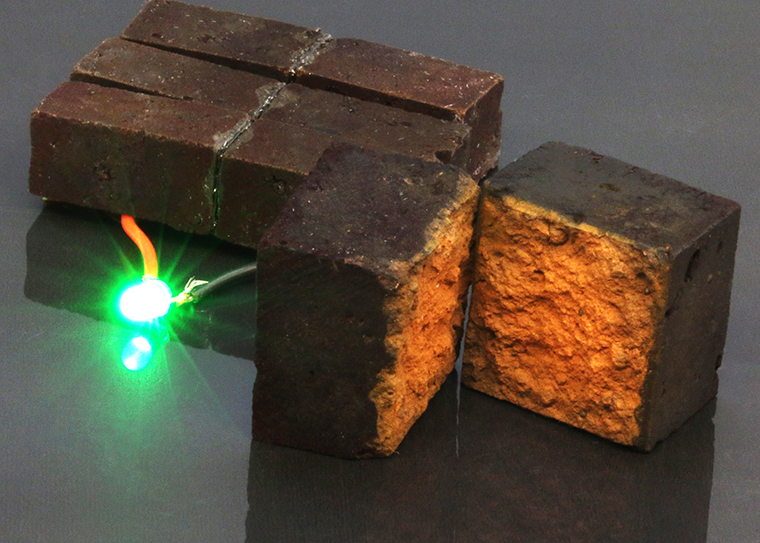
What is it? Engineers at Washington University in St. Louis devised a way to turn the common red brick — same kind as you can pick up at the hardware store — into an energy storage unit.
Why does it matter? Buildings whose walls have the ability to charge a phone or a computer or supply electricity to a light fixture have an obvious appeal, and the researchers imagine their creation could be useful in, for instance, emergency lighting situations, perhaps when connected with solar cells. And as they point out in their article in Nature Communications, fired red brick is “a universal building material” whose use dates back 5,000 years. That’s a lot of potential batteries.
How does it work? The Washington University team developed the energy storage device by creating “a coating of the conducting polymer PEDOT, which is comprised of nanofibers that penetrate the inner porous network of a brick; a polymer coating remains trapped in a brick and serves as an ion sponge that stores and conducts electricity,” explains chemistry professor Julio D’Arcy.
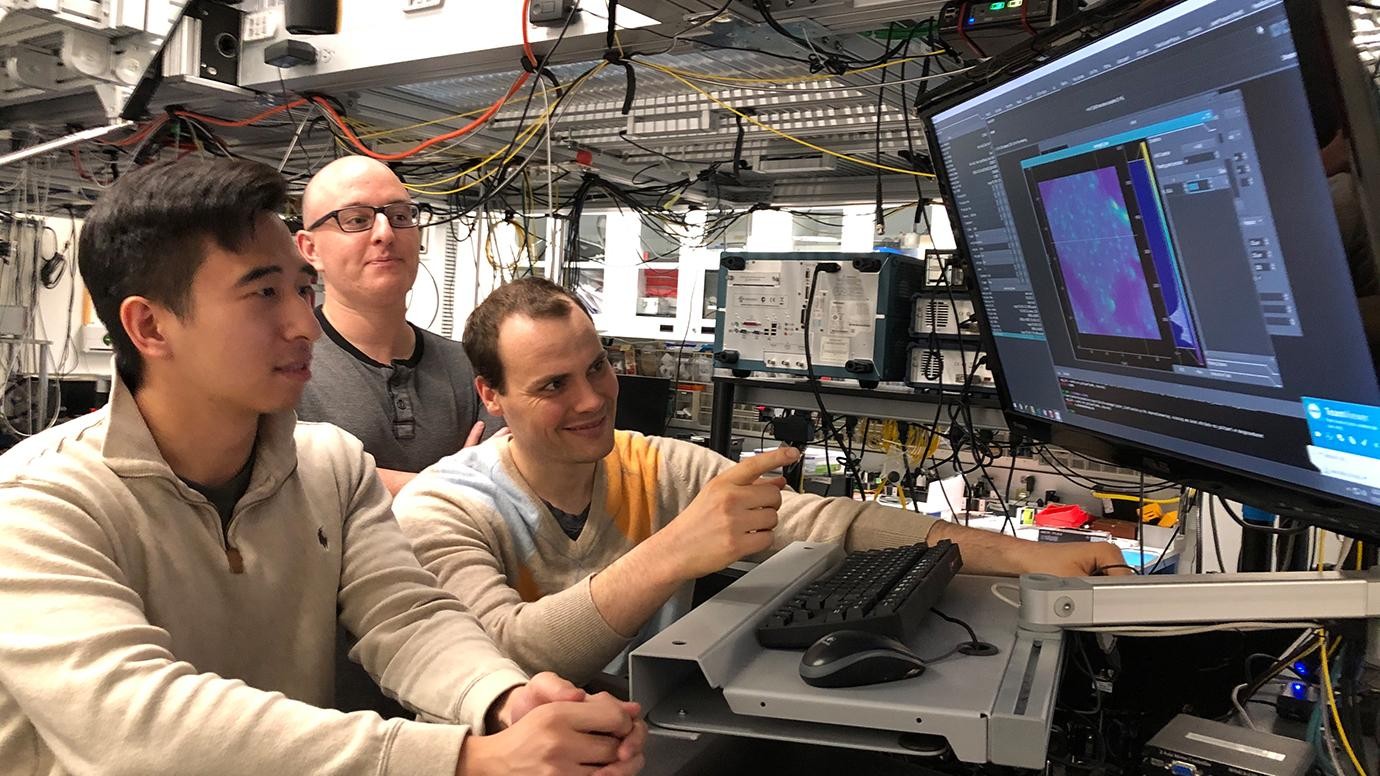
What is it? Scientists at the University of Chicago’s Pritzker School of Molecular Engineering discovered a “simple modification” that enables quantum systems to operate 10,000 times longer than before.
Why does it matter? Business and governments have eyed quantum computing as a way to create “virtually unhackable networks or extremely powerful computers,” even a quantum internet. But they have been held back by the fragility of quantum systems, which require extreme stability. Such systems now operate on the order of milliseconds. The U. of C. discovery points the way forward, said David Awschalom, lead author of a new study in Science: “This breakthrough lays the groundwork for exciting new avenues of research in quantum science. It enables new research opportunities previously thought impractical.”
How does it work? By, essentially, “tricking” the quantum system into thinking there’s no background noise, using electromagnetic pulses in addition to a precisely tuned continuous alternating magnetic field. Postdoctoral researcher Kevin Miao said, “To get a sense of the principle, it's like sitting on a merry-go-round with people yelling all around you. When the ride is still, you can hear them perfectly, but if you're rapidly spinning, the noise blurs into a background.”
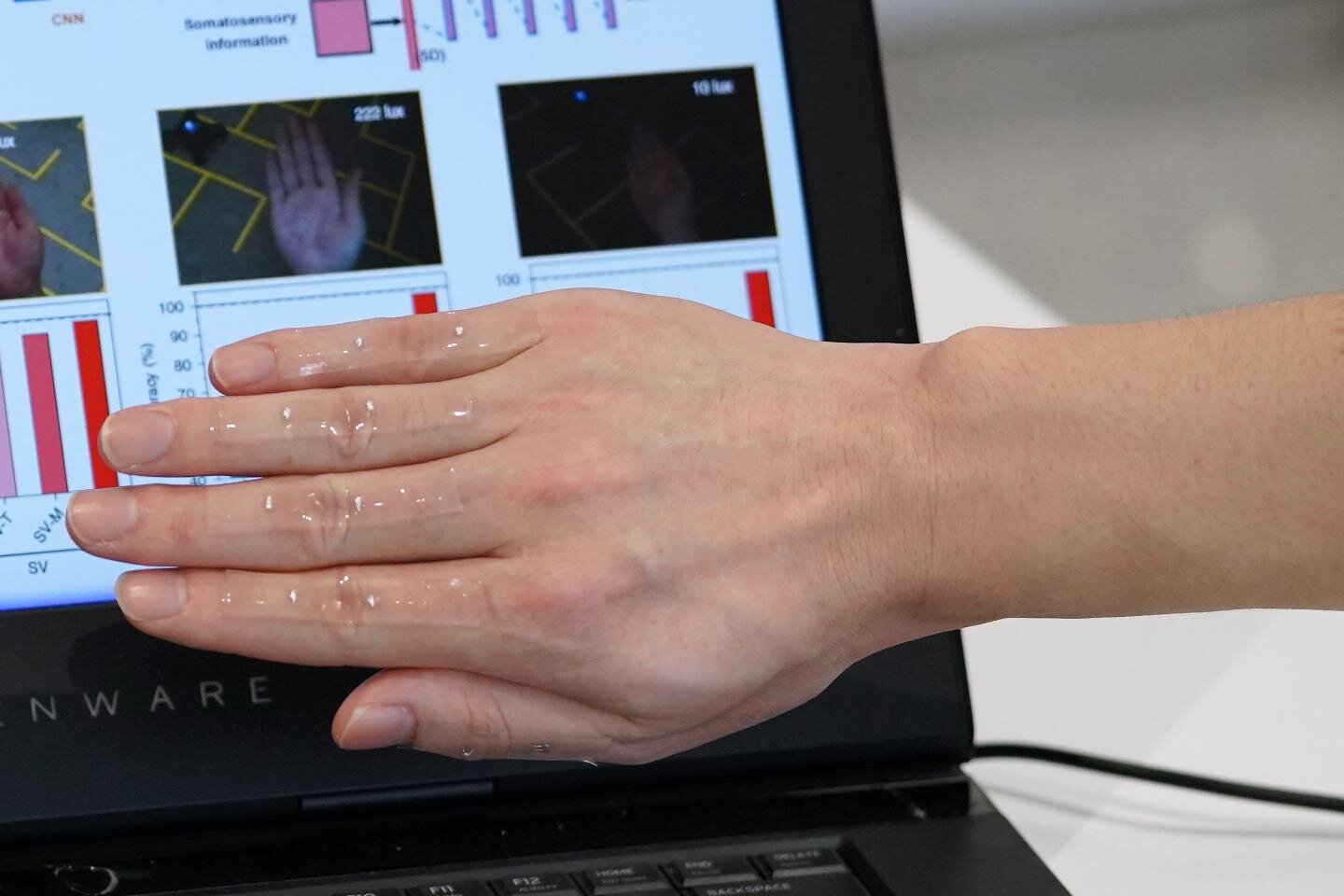
What is it? Scientists at Singapore’s Nanyang Technological University combined “skin-like electronics with computer vision” into an artificial intelligence system that can recognize hand gestures.
Why does it matter? The technology could have uses in surgical robots, gaming interfaces, and robot-aided workplaces. Markus Antonietti, the director of Germany’s Max Planck Institute of Colloids and Interfaces — who was not involved in the project — said in NTU’s press release that “the findings from this paper bring us another step forward to a smarter and more machine-supported world. Much like the invention of the smartphone, which has revolutionized society, this work gives us hope that we could one day physically control all of our surrounding world with great reliability and precision through a gesture.” The paper was published in Nature Electronics.
How does it work? The Singaporean team’s “bio-inspired” system includes a stretchable sensor, made of single-walled carbon nanotubes, that fits over the hand, while the AI system combines three different neural network approaches: one concerning visual processing, one concerning somatosensory processing and one that fuses the two. NTU’s Chen Xiaodong, the study’s lead author, said the technology is “unique” in that it resembles “the somatosensory-visual fusion hierarchy in the brain.”
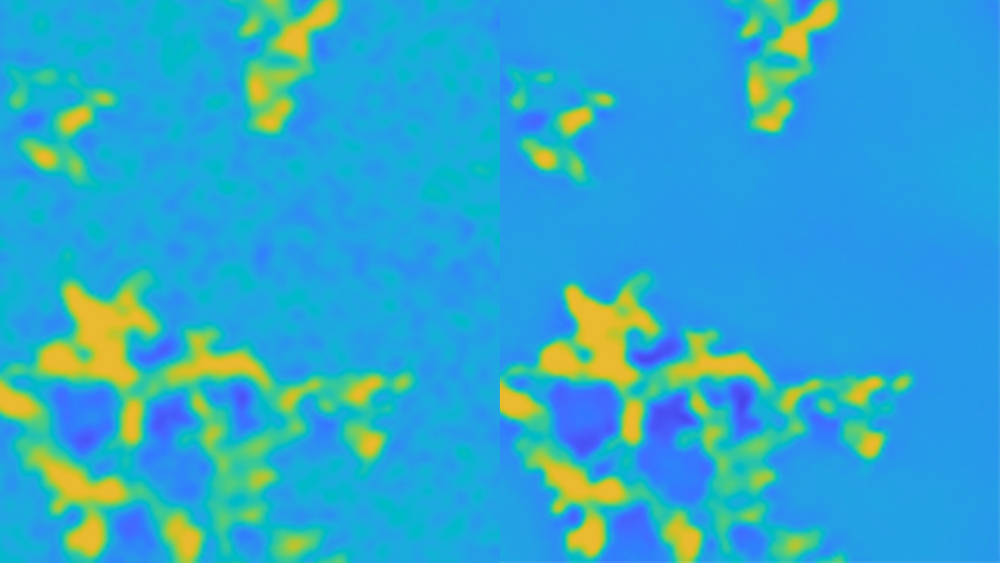
What is it? Researchers at Texas A&M University are working on a new method that uses machine learning to improve the quality of low-resolution images produced by electron microscopes.
Why does it matter? The technique may have solved an old problem in electron microscopy, which — as the name suggests — obtains images by means of a high-energy electron beam aimed at the sample. Higher resolution can be achieved by cranking up the energy, only this can damage the sample under examination, similar to how ultraviolet rays can damage the skin. “There's always that dilemma for scientists,” said engineering professor Yu Ding, who co-authored an article on the technique in IEEE Transactions on Image Processing. “To maintain the specimen's integrity, high-energy electron beams are used sparingly. But if one does not use energetic beams, high-resolution or the ability to see at finer scales becomes limited.”
How does it work? Ding and colleagues trained a neural network on pairs of images at low and high resolutions, which enabled the AI to learn how to enhance details on other low-res images. Ding explained, “Normally, a high-energy electron beam is passed through the sample at locations where greater image resolution is desired. But with our image processing techniques, we can super-resolve an entire image by using just a few smaller-sized, high-resolution images. This method is less destructive since most parts of the specimen sample needn't be scanned with high-energy electron beams."