Tiny Carbon Filters To Quench Man's Thirst For Desalinated Water
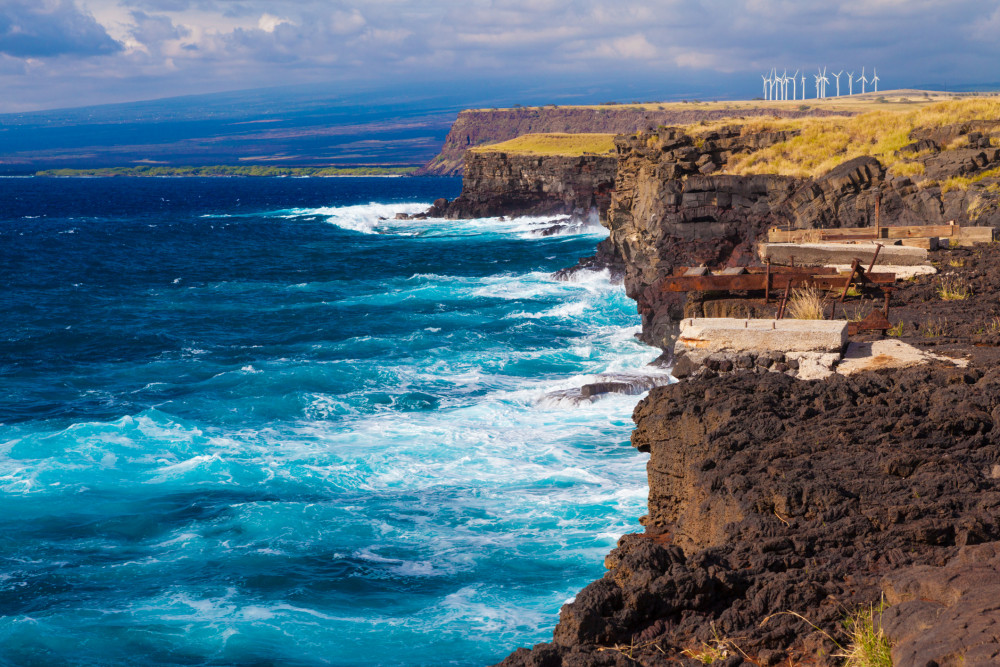
What is it? Researchers at Northeastern University and Lawrence Livermore National Laboratory have found a way to use carbon nanotubes — microscopic tubes, a fraction of the size of a human hair — to filter salt out of water. The new method could provide cheap, drinkable water to people around the world.
Why does it matter? Only a fraction of the earth’s water is fit for human consumption; most is full of salt. Desalination — the process of removing salt from water — is expensive and uses too much energy to be done on a large scale. But according to the new study, filtration through carbon nanotubes could be done in a cost-effective way, at scale.
How does it work? Carbon nanotubes can be made narrow enough to allow only one water molecule through at a time, a process that would help break the bonds between water and salt. "You can imagine if you're a group of people trying to run through the hallway holding hands, it's going to be a lot slower than running through the hallway single-file," said Meni Wanunu, one of the researchers. Also, the nanotubes are negatively charged, which causes them to reject other negatively charged particles, like salt. The combination of these two processes — filtration and negative charge — enables quick, inexpensive filtration.
Lobster Helmets
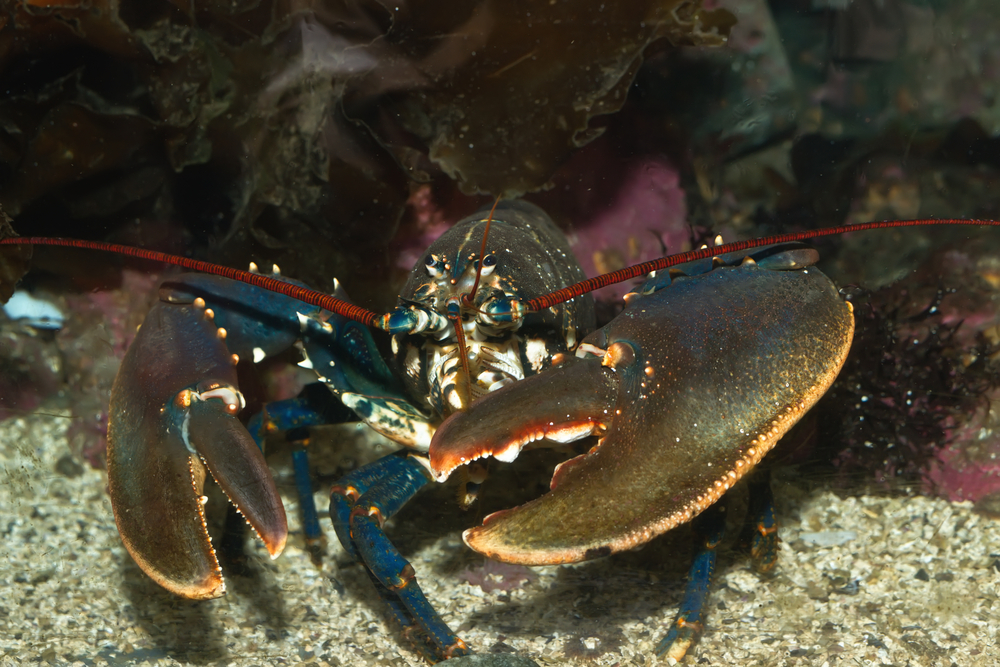
What is it? Researchers at the University of Southern California have developed a new material, inspired by lobster shells, that is eight times stronger than conventional plastic. They believe it could revolutionize the way we protect football players.
Why does it matter? Every year, football players suffer thousands of concussions, with devastating long-term consequences. A recent study of the brains of deceased football players found that 99 percent suffered from chronic traumatic encephalopathy, a condition caused by repeated head injuries that’s associated with a number of problems, including personality changes, loss of motor skills and Parkinson’s disease. USC’s new plastic could enable stronger helmets, adding an extra level of protection against CTE. It also offers new hope for players who have already been injured: The research team has used it to construct an artificial meniscus, or knee cartilage — a body part that’s often injured during sports.
How does it work? In lobster shells, fibers align in a Bouligand structure, a spiral design that makes it hard for cracks to expand. “You may have a microcrack, but it doesn’t break the shell,” explains Yong Chen, a member of the USC team. To imitate it, researchers added carbon nanotubes to the plastic in a 3D printer, then used an electric charge to arrange them into a Bouligand framework. This is the first time that researchers have integrated an electrical field into 3D printing, and Chen thinks the process could revolutionize a host of industries, including aerospace, mechanical, and tissue engineering.
A Vaccine That Could Help Addicts Quit Opioids
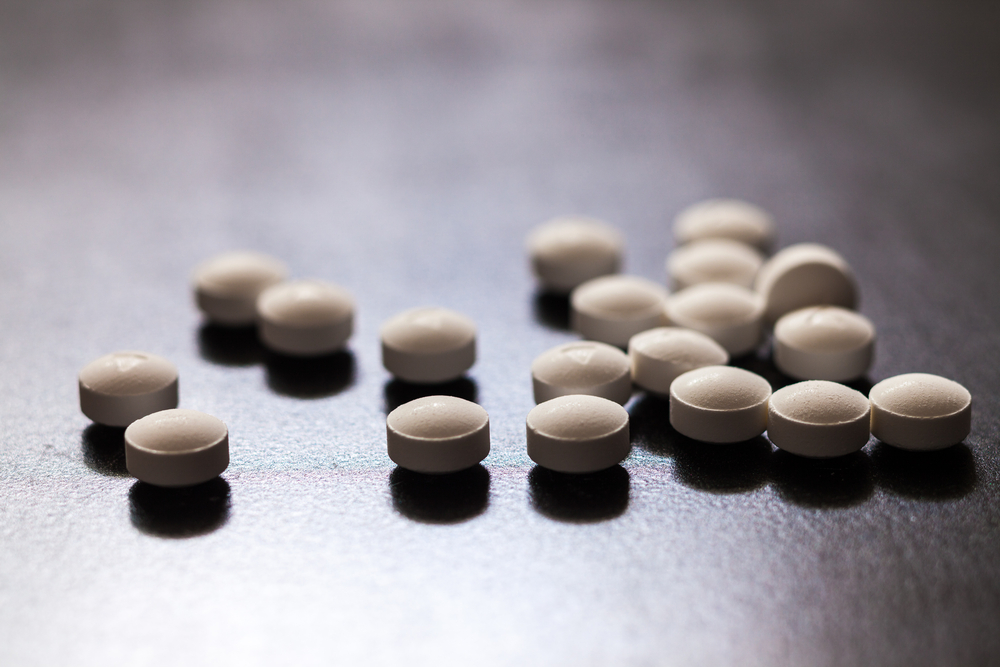
What is it? Scientists at the Scripps Research Institute in California have developed a vaccine that counters the effects of heroin and fentanyl, two commonly abused opioids. Ultimately, they believe, it could lead the way to protecting against opioid overdoses.
Why does it matter? Opioids caused an estimated 60,000 deaths in the U.S. last year. And synthetic opioids like fentanyl, commonly prescribed for pain, make the problem worse. "We'll see more problems going forward, simply because it takes a couple of months to grow poppies, but it only takes a couple of days to make the synthetic opioid," says Kim D. Janda, a member of the Scripps team. Most antidrug efforts have focused on controlling access to drugs, a strategy that is hamstrung by the sheer numbers of opioid prescriptions written every year. The Scripps vaccine, on the other hand, could render addicts immune to the effects of opioids, making it easier for them to quit.
How does it work? Historically, making an opioid vaccine has been impossible because the human body doesn’t recognize opioid molecules as a threat. Janda’s team created a new molecule, called a hapten, that imitates opioids, but which can be attacked by antibodies. It teaches the immune system to recognize opioids so that later, when faced with real opioids, it will deliver antibodies that bind to the drugs and prevent them from crossing the blood-brain barrier. The researchers estimate that their vaccine — which so far has only been tested on mice and monkeys — would work for eight months.
Mini Antennae Today, Bio-implantable Antennae Tomorrow?
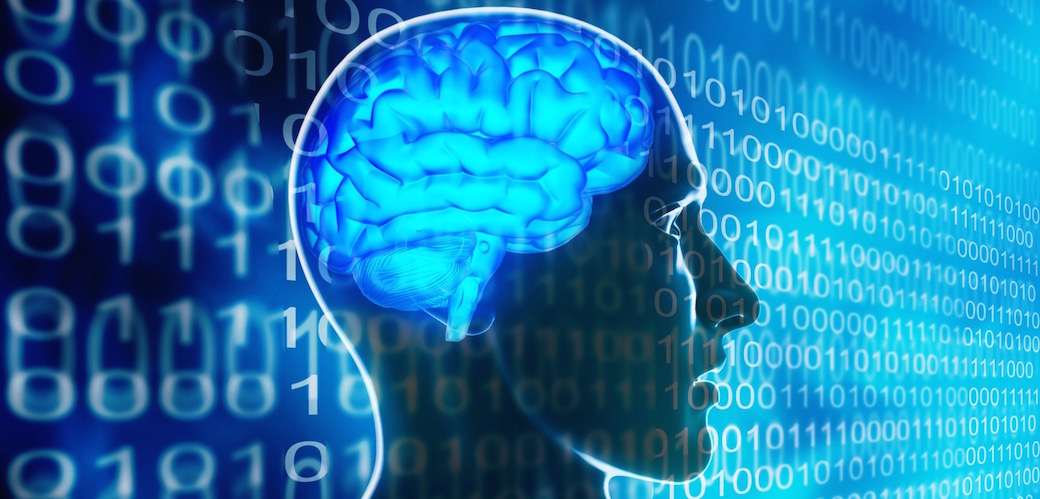
What is it? Researchers at Northeastern University have found a way to make wireless antennae that are 100 times smaller than the current models, a move that could revolutionize wireless communication.
Why does it matter? Right now, cellphones and other wireless devices use fairly large antennae. Smaller antennae could open the door to smaller devices — cellphones that could fit on a finger, for example, or medical devices that could be implanted in your brain. “[It’s] like science fiction,” says Nian Sun, the study leader, who hopes to develop sensors that could treat epilepsy and other neurological disorders.
How does it work? Antennae have to be roughly as long as the waves they interact with, so traditional antennae, which are designed to pick up large electromagnetic waves, tend to be large. However, by converting electromagnetic waves to much smaller acoustic vibration waves, Sun’s team has made it possible to build antennae that are millimeters in size, but which pack the receiving power of full-size electromagnetic antennae.
Turning Autumn Leaves Into Advanced Electronics
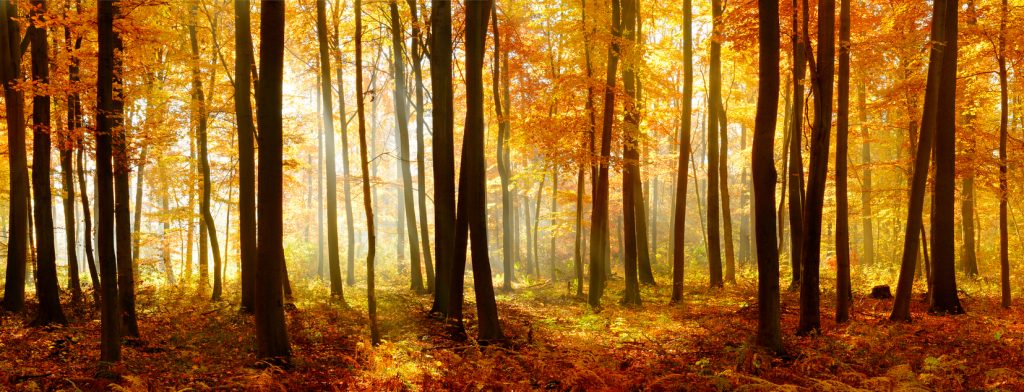
What is it? Researchers at China’s Qilu University of Technology have found a way to convert dead tree leaves into supercapacitors, electronic components that can store huge amounts of energy.
Why does it matter? In China, dead leaves are routinely collected and burned, creating clouds of smoke and adding to the country’s already substantial air pollution. The new process could help alleviate that problem. What’s more, the supercapacitors that it produces could be the key to creating the next generation of electric cars, a move that would further improve air quality in China — and around the world.
How does it work? Researchers ground the leaves into a powder, heated them and treated them with chemicals, ultimately producing highly porous carbon microspheres. These little balls have an extraordinary amount of surface area, a characteristic that enables them to store large amounts of electricity. Currently, the Qilu team is experimenting with other preparation techniques to see if they can further increase the microspheres’ energy-storage capabilities.